Research and chairs
The list contains presentations of research facilities, institutes and chairs for nuclear technology in Germany. Both internationally networked research at a high level and training in the field of nuclear technology are possible there.
University of Stuttgart - Institute for Nuclear Energy and Energy Systems (IKE)
Acquiring and developing the skills of students and doctoral candidates is the guiding principle of the staff at the Institute of Nuclear Energy and Energy Systems (IKE) at the University of Stuttgart. In a mix of classic nuclear energy technology, reactor safety research and innovative methods, the audience is introduced to the "fascination of nuclear technology".
Initial situation for nuclear technology in Germany
"Do we still need nuclear engineering chairs and research into reactor safety? Hasn't this been done with the shutdown of the last power reactors? I'm getting these questions a lot again at the moment," explains Prof Jörg Starflinger, Managing Director of the Institute of Nuclear Power and Energy Systems at the University of Stuttgart. "And my answer is always: Yes, of course we need it!" The background to this assessment is the shortage of skilled labour, which is also clearly noticeable in academia. The number of engineering students at the University of Stuttgart is declining significantly. A steady stream of graduates is needed to fill vacancies in the nuclear industry. Decommissioning and dismantling will certainly continue to be important topics for another 30 years. From a strategic perspective, a viable national concept for the further development of skills in nuclear technology is required to assess the development of new plants in our neighbouring European countries. In the long term, relevant expertise that cannot simply be stored in databases must be continued and constantly developed in line with the current international state of research and science until a final repository is closed. The latter in particular is a societal, cross-generational task that must be mastered jointly by all key players. This also requires the continuous training and development of future experts with the involvement of research and development, industry, expert organisations and supervisory authorities. The IKE is available for this important social task.
Nuclear technology teaching
Teaching consists of the fundamentals of nuclear technology and further specialisations. In addition to the lectures Nuclear facilities for power generationin which the structure and function of nuclear power plants (incl. Gen III+, Gen IV, SMR[1] and MMR[2]- annexes), students can improve their knowledge in the lectures Reactor physics and safety, Modelling of nuclear facilities and Radiation protection deepen. In Probabilistic and Monte Carlo methods students are familiarised with current methods for sensitivity and uncertainty analyses, such as those used in the GRS code SUSA. Interestingly, this lecture is attended by many aerospace engineering students at the University of Stuttgart, who see it as a valuable addition to their curriculum.
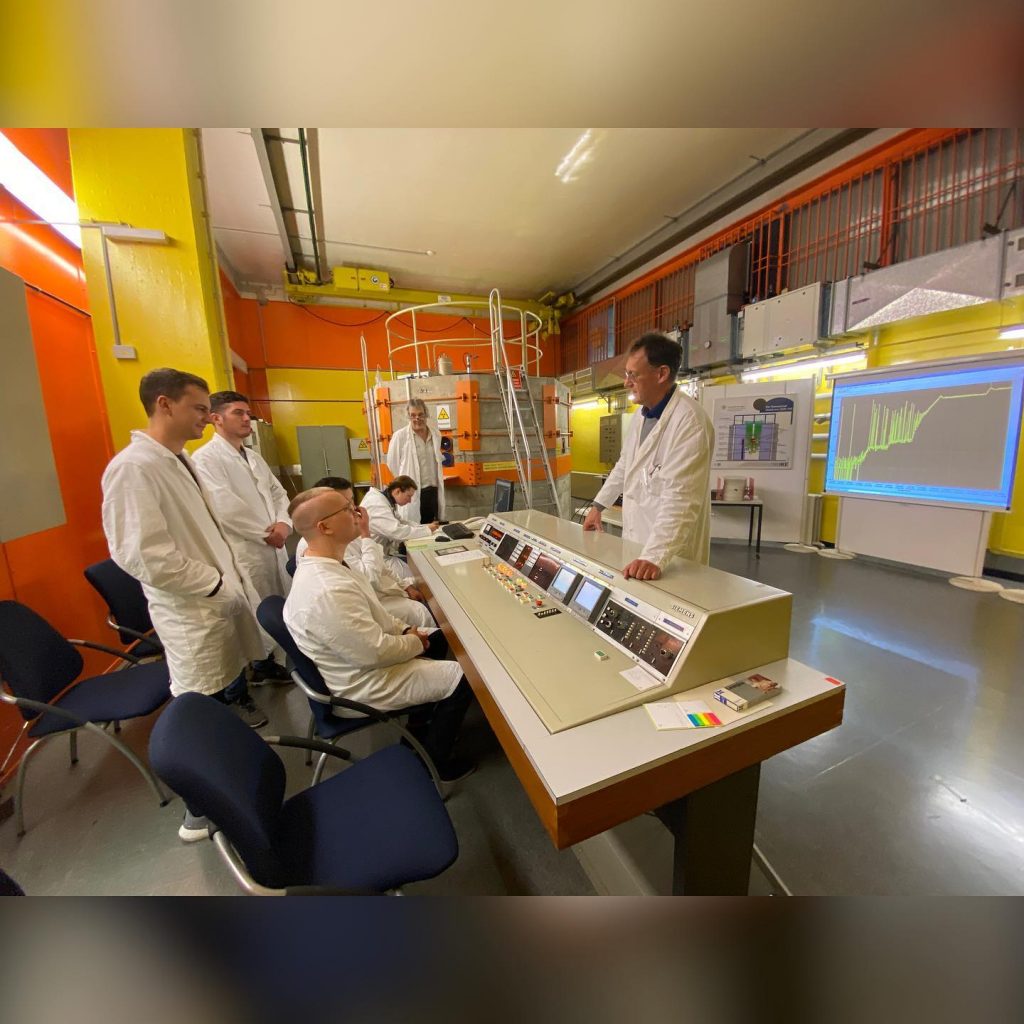
Internship at the SUR-100 of the University of Stuttgart. Photo: Nelson Rincon, IKE
A new addition is the lecture Nuclear Wastefor the English-language WASTE degree programme and as an offer for students of environmental engineering. Furthermore, a German-language lecture Nuclear waste - where to put it? for non-MINT students[3] was offered for the first time last winter semester. Students of history and architecture were the first participants in this lecture, which will be expanded and, if necessary, will inform academically interested people outside the university about radioactive waste, disposal and final storage concepts in the "Studium Generale".
As a teaching export, the IKE provides biomedical engineering students at the universities of Tübingen and Stuttgart with knowledge about Radioactivity and radiation protection and Basics of medical radiation technology. The latter lecture serves as an introduction to the lecture "Fundamentals of therapy with ionising radiation", which is held by the medical physicist of the Robert Bosch Hospital and the Marienhospital Stuttgart.
Internships at the Siemens teaching reactor (SUR-100)
All degree programmes include internships. With its Siemens teaching reactor (SUR-100), a zero-power reactor with a rated output of 100 mW at the Stuttgart site, the IKE offers a wide range of practical courses.[4]practical experiments on a nuclear reactor. For example, the neutron flux curve in the SUR can be deduced on the blackboard or measured directly on the reactor. This type of experimental set-up is the ideal complement to the blackboard and therefore supports learning success. Furthermore, activation experiments can be carried out and, for example, half-life times can be determined. After activation in the SUR-100, the gamma spectrometer can be used to identify the ingredients of substances. For example, students repeatedly discover silicon, copper, gold and silver in the integrated circuits of the 1980s. One current project is an experimental set-up for the production of technetium 99 from molybdenum 98. The aim is to provide medical technology students with a practical explanation of how this important radiopharmaceutical is produced. The learning success with these practical experiments is much greater than with theory alone.
Innovative research topics
According to Humboldt's educational ideal, teaching and research at a university are inextricably linked. In keeping with this ideal, research work is carried out at the IKE on very current topics, of which only two examples should be mentioned here: Passive heat dissipation and artificial intelligence. All topics are usually dealt with by doctoral students who build up or improve their knowledge and skills by working on the scientific topics. This makes them very interesting for the labour market, unfortunately often also outside of nuclear technology. Project funding is provided by the federal ministries and the EU, not by industrial contracts.
Passive residual heat removal has become the focus of reactor safety research as a result of the accident in Fukushima. This raises the question of how residual heat can be dissipated in the event of an emergency and residual heat removal chain failure and a destroyed infrastructure (no access for external measures). An EU-funded project is evaluating the possible use of a self-sufficient, self-starting, very compact, retrofittable residual heat removal system with supercritical carbon dioxide as a working fluid (sCO2-4-NPP[5]). For a generic KONVOI, it was shown that 4 such circuits can reliably dissipate the decay heat. Another project is the passive cooling of wet storage facilities using heat pipes (PALAWERO 2, BMWi (now BMUV), funding code: FKZ 1501515). The liquid in the heat pipe evaporates in the evaporation zone and transports the heat to the condensation zone, usually at the upper end of the heat pipe, in order to utilise buoyancy forces during evaporation. The heat is released into the ambient air, for example, which reduces the dependency on water storage. So-called. Loop heat pipes can also be used for safe passive residual heat removal in new SMR designs (see EU project PASTELS[6]). More examples can be found on the IKE website: www.ike.uni-stuttgart.de/forschung/forschungsprojekte
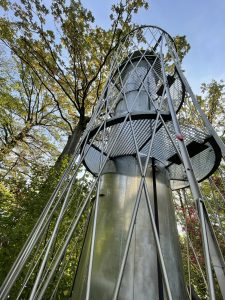
Chimney of the ATHOS test facility (Atmospheric THermosyphon COoling System). Photo: Nelson Rincon, IKE
Artificial intelligence (AI) is another future topic in nuclear technology. This is less about the formulation of texts[7]but how an AI can be trained so that highly complex, computationally intensive simulation processes, such as the late incident phase, can be carried out with a reasonable amount of resources. Not everyone has access to a mainframe computer. In addition to the IKE, the Institute for Parallel and Distributed Systems at the University of Stuttgart and the Plant Simulation and Safety working group at the Ruhr University Bochum have joined forces to derive models (so-called surrogate models) from a very extensive database, which both nuclear technology institutes possess, with the help of AIs, which are favourable in terms of computing time but validated by the amount of data, and which can then be used in thermohydraulic system codes, such as ATHLET from GRS. The project is funded by the BMBF (FKZ: 02NUK078). In addition to the scientific objective, the focus here is also on developing the skills of doctoral students, who are supported by dedicated students.
Future in research and teaching?
Consider the following question: Given that there may not be a final repository for heat-generating waste until 2079 or even much later (see atw 03/2023), who will actually still be carrying out a criticality analysis for the fuel elements in the CASTOR© casks in 2050 according to the (then) current state of science and technology? Knowledge cannot be stored. You store data and information. Knowledge is generated by constantly working with data and facts. Experience (expertise) is gained from knowledge that is applied correctly and, even better, incorrectly.
We generate knowledge through projects, whether national or international. Knowledge is passed on at universities and colleges by teaching students to work scientifically with facts and data. Who is supposed to do this if there is a risk that universities will close their nuclear technology institutes in line with political expectations? The very good, strategic junior researcher programmes of the BMBF and BMWi (now BMUV) with their targeted funding of junior research groups will then come to nothing. The question of "Teaching and lecturers 2030" must now be clarified with the involvement of the federal and state governments. Perhaps it is even time for a "nuclear academy"?
Prof. Dr.-Ing. Starflinger
Managing Director
Institute for Nuclear Energy and Energy Systems
Phone: +49 711 685 62138
institut@ike.uni-stuttgart.de
www.ike.uni-stuttgart.de
[1] Small Modular Reactor
[2] Micro-Modular Reactor
[3] Mathematics-Computer Science-Natural Sciences-Technology
[4] further SUR-100 sites at Ulm University of Applied Sciences and Fortwangen University of Applied Sciences as well as a similar zero-power reactor (AKR-2) at TU Dresden.
[5] https://www.sco2-4-npp.eu/ last visited on 8.6.2023
[6] https://www.pastels-h2020.eu/ last visited on 8.6.2023
[7] Although the professor who reads the students' work increasingly wishes that ChatGPT or similar had been used
University of Stuttgart Institute for Nuclear Energy and Energy Systems from atw 04-2023
Technical University of Munich - TUM Centre for Nuclear Safety and Innovation (TUM.CNSI)
TUM.CNSI's motto "Maintaining expertise through research" summarises our mission and motivation in three words. We are convinced that nuclear technology is more than just a bridging technology, that it already provides solutions to existential problems and that it will make a decisive contribution to successfully mastering the challenges of the coming decades. However, this is only possible if the current open questions are tackled by motivated research projects. We are convinced that universities are the ideal institutions for this. Nuclear technology is almost irreplaceable, particularly in the urgently needed decarbonisation process, and the same applies to the development of state-of-the-art drugs for cancer diagnostics and therapy. Active research on the one hand and the transfer of the necessary expertise to motivated students and young scientists on the other has been one of the main cornerstones of TUM.CNSI since its foundation in 2021.
Nuclear energy - taking stock
Despite the completed phase-out of the use of nuclear energy for the commercial generation of electricity in Germany, nuclear technology will continue to play an important role in Germany as a centre of science and research due to its wide range of applications. It is used in numerous areas, such as materials testing, basic research and the production of radiopharmaceuticals. A small number of research reactors and radiochemical facilities play a key role in the global supply of radiopharmaceuticals such as technetium-99m. Nuclear technology expertise is also fundamental to radiation research and radiation protection. The decommissioning and dismantling of existing nuclear facilities in Germany and the search for, qualification and commissioning of final storage sites for radioactive waste will also continue for decades to come. At the same time, the vast majority of European and international industrialised societies view nuclear energy much more favourably, particularly with regard to its positive contribution to climate and environmental protection. There are currently 50 nuclear reactors under construction worldwide.
In the first half of the 21st century and beyond, extensive expertise and application experience in the nuclear field will therefore continue to be required in Germany. In addition, it is in Germany's own security interests to have expertise in nuclear security in order to be able to actively contribute this internationally. This expertise explicitly refers not only to the preservation of knowledge already acquired. With regard to the development and implementation of new reactor concepts in other European and non-European countries, Germany must also conduct its own research in order to be able to discuss and argue internationally on an equal footing.
Garching reactors - the nuclear beginning and end in Germany
While the Munich Research Reactor (FRM) heralded the beginning of the reactor age in Germany in 1957, it is expected to come to an end towards the end of the 21st century with the shutdown of the Heinz Maier-Leibnitz Research Neutron Source (FRM II). Both reactors have had a lasting impact on the Garching site and nuclear research and teaching at TUM is historically closely interwoven with the FRM II in particular. As a conversion to a fuel with lower enrichment was planned and politically anchored from the very beginning, the High Density Nuclear Fuel / Reactor Physics working group was founded in 2003. It develops the new nuclear fuel required for the conversion and realistic, theoretical conversion scenarios. Since 2013, it has been operating a nuclear fuel laboratory, the only one of its kind in Germany, for the research and development of new, high-density nuclear fuels for research reactors. As a result of these long-standing efforts, it was demonstrated in 2022 that it is scientifically possible to convert the FRM II to a low-enriched fuel element using a new type of monolithic uranium-molybdenum fuel and geometric modifications. This lays the foundations for the long-term continued operation of the Garching neutron source.
Pooling expertise - founding of TUM.CNSI
As of this year, TUM is the only operator of a nuclear reactor in regular operation with a significant thermal output and, with Radiochemistry Munich (RCM) and the Chair of Nuclear Technology located in the immediate vicinity, also has a portfolio of nuclear technology skills that is unique for a university in Germany. It was therefore a logical step to bring together the existing expertise on campus under a common name, so that in 2021 the TUM Centre for Nuclear Safety and Innovation (TUM.CNSI) was founded. The expertise available at TUM is bundled into thematic areas so that research projects can be dealt with on an interdisciplinary basis. These include, for example, the development of new reactor concepts, new solutions for the processing and disposal of nuclear waste and the development of new medical radioisotopes.
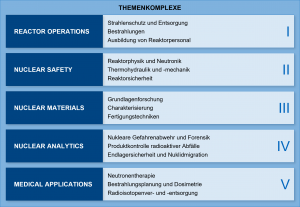
Thanks to the expertise of the participating institutes, TUM.CNSI covers a wide range of nuclear technology topics.
Unrivalled infrastructure - laboratories and computing clusters
A major focus of TUM.CNSI at the FRM II is on applied research, which is carried out in various laboratories. In particular, research into monolithic uranium-molybdenum fuels (U-Mo) is currently a central research topic, as only these have the necessary uranium density to convert the FRM II to low enriched uranium (LEU). The nuclear fuel laboratory has several gloveboxes in which uranium in various forms can be handled openly. The research work is carried out in close co-operation with national and international partners and, although motivated in particular by the conversion of the FRM II, there are also other potential application scenarios, e.g. for so-called Small Modular Reactors (SMRs). Furthermore, the experimental capacities of TUM.CNSI are being continuously expanded. For example, the nuclear fuel laboratory has been significantly upgraded by doubling its floor space, and its analytical capabilities have also been significantly improved by the installation of an FIB-SEM with EDX and EBSD detectors, among other things. An application is currently being prepared to extend the licence to include the handling of thorium, which can be used as fuel for various alternative reactor concepts.
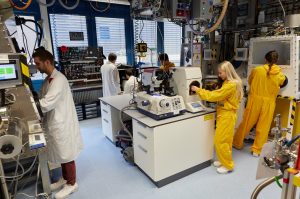
By working on new fuels as part of the FRM II upgrade, students can experience nuclear research at first hand in the nuclear fuel laboratory. Bernhard Ludewig, FRM II / TUM
The second, central pillar is the theoretical development of new reactor models, such as an LEU core for the FRM II. State-of-the-art computer programmes are used here, such as Serpent 2 for neutronics, Stromfaden and Computational Fluid Dynamics (CFD) codes for thermo-hydraulics as well as various mechanics programmes. TUM.CNSI has several computer clusters with a total of 2900 CPUs and a GPU cluster at its disposal in order to be able to carry out the necessary calculations and to take the size of the group into account. Here too, the capacities of both systems are currently being significantly expanded.
The conversion of the FRM II, but also new reactor concepts, benefit from the qualification of CFD programmes for nuclear applications. The gold standard for this is the comparison of theoretical calculations with experimental data. The latter are only available in very limited quantities for high-performance research reactors, which is why a second laboratory is currently being set up for the specific purpose of validating calculation methods. In this laboratory, a new hydraulic test rig is being set up in which heat transfer phenomena and turbulence models can be investigated under reactor conditions. In addition, TUM.CNSI has access to the hydraulic laboratory, the research reactor and the hot cells at McMaster University in Hamilton, Canada, and can thus round off the experimental portfolio.
Teaching and research as a guarantee for expertise
Knowledge and expertise can only be maintained through active research and training. TUM.CNSI and its individual partners have been making a significant contribution to this at TUM for years. The lectures in particular, which are supervised by TUM.CNSI, attract many students to nuclear research. The Reactor Physics I & II lectures are particularly successful, each with more than 50 students. Both lectures are complemented by the other courses "Radiation and radiation protection", "Introduction to nuclear energy", "Fundamentals and thermohydraulic analysis of power plants" and "Fundamentals of nuclear technology". The diverse range of topics that had and still have to be dealt with as part of the conversion of the FRM II makes this one of the most successful programmes for nuclear technology training in Germany. By awarding theses, TUM.CNSI can also offer students long-term and, above all, application-related training opportunities. Students also benefit from the close cooperation with Canada and its active civil nuclear programme. Through the exchange, students can, for example, participate in the development and construction of an SMR or in irradiation experiments.
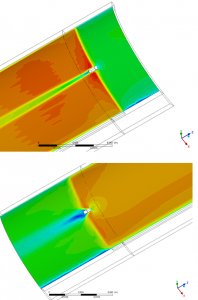
Exemplary result of a CFD calculation for a FRM II fuel plate
Future challenges: Funding and distribution of subsidies
The so-called "nuclear phase-out" also poses major challenges for TUM.CNSI. In particular, the reduction in available funding and its uneven distribution to those involved in nuclear technology raises the question of whether the excellent conditions that have characterised research and teaching at TUM in the past can be preserved for the future. This question is of an existential nature and requires courageous and swift action on the part of all those involved. However, due to the existing support, we are optimistic that a sustainable answer will be found and that nuclear research and development at TUM can not only be secured, but also expanded. Thanks to the facilities present on the Garching campus, TUM has all the prerequisites to maintain its outstanding position in nuclear technology education in Germany in the future.
CONTACT
Heinz Maier-Leibnitz Research Neutron Source (FRM II)
Technical University of Munich
Lichtenbergstr. 1, 85748 Garching
www.frm2.tum.de
Dr rer. nat. Tobias Chemnitz
tobias.chemnitz@tum.de
Dr rer. nat. Christian Reiter
Christian.Reiter@frm2.tu-muenchen.de
The Karlsruhe Institute of Technology (KIT)
The Karlsruhe Institute of Technology (KIT) is "The Research University in the Helmholtz Association". As the only German university of excellence with large-scale national research, we offer our students, researchers and employees unrivalled learning, teaching and working conditions. The roots of the university education centre go back to the year 1825. KIT took its present form when the University of Karlsruhe (TH) and the Karlsruhe Research Centre merged in 2009.
The Nuclear Waste Management, Safety and Radiation Research Programme (NUSAFE) at KIT is part of the NUSAFE programme in the Helmholtz Association's Energy research field and stands for societal precautionary research into nuclear safety.
The Safety assessment of nuclear reactors and the Protection of the population and our environment from radiation exposure are strategic, long-term goals of NUSAFE precautionary research - even after the end of nuclear power generation in Germany. Internationally, nuclear energy has a long-term perspective: countries such as China, South Korea, Japan and the USA are building new nuclear power plants, and European neighbours such as France and Finland are also continuing to rely on nuclear energy.
Disposing of radioactive waste responsibly and storing it safely in a repository will remain a challenge for a very long time. The Safety of a repository system must be proven by law for a period of one million years. The NUSAFE programme conducts research into the final disposal of radioactive waste. One focus is on fundamental processes that have a relevant influence on long-term safety. We are investigating how radioactive waste changes and behaves over time and how so-called radionuclides - i.e. radioactive elements - can be safely stored in repositories. We are also investigating which steps are still required: What needs to be considered when dismantling nuclear facilities? How should problematic (special) waste types be handled? What additional issues arise from the foreseeable extended interim storage of spent fuel elements? How should nuclear material monitoring be organised?
In order to answer these questions, we Unrivalled laboratory infrastructure and thus create the necessary conditions for excellent nuclear safety research. We are also intensively dedicated to Training and promotion of young scientists and technicians, which is urgently needed by authorities, industry and science.
The lectures offered at KIT in the field of nuclear safety research are briefly described below.
The Institute for Neutron Physics and Reactor Technology (INR) sees itself as an international institute for energy research. Innovation and research include fusion technology, solar thermal energy, thermal storage, thermoelectric conversion concepts and safety analyses of nuclear facilities from accelerators to power plants.
The INR offers the following lectures in the field of nuclear technology:
Nuclear power plant technology:
The aim of the course is to qualify students for a research-related career in nuclear power plant engineering. Participants will be able to describe the most important components of nuclear power plants and their function. They will be able to design or modify nuclear power plants independently and creatively. They acquire a broad knowledge of this power plant technology, including specific knowledge of core design, the design of primary and secondary systems and nuclear safety technology. Based on the thermodynamics and neutron physics they have learned, they will be able to describe and analyse the specific behaviour of nuclear power plant components and assess risks themselves. Participants in the lecture have trained analytical thinking and judgement in the construction of nuclear power plants.
In a further lecture module, innovative nuclear systems:
The aim of the lecture is to convey the current status and development directions of nuclear technology. Nuclear systems that have good prospects from today's perspective are presented. The main characteristics of such systems and the associated challenges are presented and discussed. This includes the current status and development trends in nuclear technology as well as advanced concepts of the water-cooled reactor, fast reactors, which can also be used as transmutation systems for the treatment of nuclear waste, and development directions of the gas-cooled reactor, as well as fusion systems.
In the lecture Energy Systems II the Fundamentals of reactor physics mediated:
Students acquire comprehensive knowledge of the physics of nuclear fission reactors: Neutron flux, cross sections, fission, breeding processes, chain reaction, critical size of a nuclear system, moderation, reactor dynamics, transport and diffusion equation for the neutron flux distribution, power density distributions in the reactor, one-, two- and multi-group theories for the neutron spectrum. Based on their knowledge of reactor physics, students will be able to understand, compare and evaluate the capabilities of different reactor types - light and heavy water reactors, Generation IV nuclear power plants - and their basic nuclear safety concepts. Students are qualified for further education in the field of nuclear energy and safety engineering as well as for (also research-related) professional activities in the nuclear industry.
In a further lecture, the Fundamentals of reactor safety mediated:
The lecture discusses the basic principles and concepts of reactor safety including methods for safety assessment and severe core-destructive reactor accidents.
The aim of the lecture is to convey the basics of reactor safety, which are required to assess the safety of nuclear facilities and the evaluation of reactor accidents such as Chernobyl and Fukushima. Starting with an explanation of the main systems of a nuclear power plant, the safety systems and concepts of different reactor types are discussed. The development and progression of accidents and incidents and the methods used to assess them are described in detail. The Fukushima accident is then analysed, its radiological consequences are presented and the countermeasures to minimise the consequences of such accidents are discussed. Finally, new developments in the safety of third and fourth generation reactors are presented.
The Institute for Applied Materials (IAM) pursues an interdisciplinary approach to materials research that covers the diversity of materials science issues across several scales. Together with national and international partners, it researches materials from their atomic structure to their function in the product, bridging the gap between material development, process technology and system integration. The IAM has broad methodological expertise in the areas of production and processing, characterisation and simulation. The IAM organises teaching on the degree programme Materials science and materials engineering and provides materials science training for other engineering and natural science degree programmes.
On Institute for Applied Materials - Applied Materials Physics (IAM-AWP) the following lecture is offered, which also has a strong reference to nuclear safety.
Use of materials at high temperatures
The course content covers the diverse areas of application and requirement profiles for high-temperature materials. The basics of high-temperature oxidation and the influences of the gas atmosphere on high-temperature corrosion behaviour are taught, and protective measures against this form of corrosion are demonstrated. Complex mechanical stresses at high temperatures are also explained. The various high-temperature materials are also covered: Steels, Ni-based alloys, Co-based alloys, ODS alloys, refractory alloys as well as ceramics and composite materials.
On Institute for Applied Materials - Mechanics of Materials and Interfaces (IAM-MMI) Another lecture, also for nuclear engineering students, deals with the design of highly stressed components.
The content of the lecture includes the rules of common design rules, classical material laws of elasto-plasticity and creep as well as service life rules for creep, fatigue and creep-fatigue interaction.
Students will be able to name the rules of common design regulations for the assessment of components that are subject to high thermo-mechanical and/or irradiation loads during operation. They will understand which material laws are used in the state of the art and the state of research to estimate the deformation and damage occurring under these loads and to predict the expected service life. They will have an insight into the use of these generally non-linear material laws in finite element programmes and will be able to assess the key points that need to be taken into account.
The Institute for Nuclear Waste Management (INE) is primarily concerned with safety research into the final disposal of radioactive waste, focussing on repository systems/components, radiochemistry and the speciation of radionuclides. The research portfolio also includes work on the safe dismantling of nuclear facilities and geoenergy.
A radiochemistry module has been set up for Master's students, consisting of basic and advanced lectures on radiochemistry as well as laboratory courses. The focus of the lecture "Radiochemistry I" and "Radiochemistry II" focuses on basic and applied radiochemistry. The aim of the lecture is to convey the fundamentals of radio- and nuclear chemistry in order to deepen the knowledge of radioactivity with regard to the associated theory and application.
This basic lecture is supplemented by the lecture on "Chemistry of the f elements" and the lecture "Instrumental Analytics„.
In addition to the lectures, students in the radiochemistry module and students at Heidelberg University will attend a joint Three-week block internship in the Technology and Environment Training Centre and in the INE control area.
In addition, the INE, together with the INR, organises the lecture: Selected problems of applied reactor physics with exercises offered.
The following topics are covered for the students: Radioactive transformations of atomic nuclei, nuclear processes, nuclear fission and delayed neutrons, basic concepts of the cross section, basic principles of the chain reaction, static theory of the monoenergetic reactor, introduction to reactor kinetics and a nuclear physics practical course.
The department "Dismantling of conventional and nuclear structures" of the Institute for Technology and Management in Construction (TMB) deals with the research and development of dismantling technologies.
The institute's research focuses on the entire field of mechanical engineering in construction and construction operations. By additionally taking into account the special features of nuclear technology, the expertise of all sectors is pooled. Here, scientific depth and proximity to practice complement each other synergistically. The following lecture modules are offered specifically for students interested in this subject area:
- Environmentally friendly and recyclable dismantling of buildings. This course is designed to teach students how to independently plan, apply for and implement demolition, dismantling and disposal work for structural and technical facilities on site. This includes the legal, technical and practical aspects, starting with the criteria for the appropriate procedures and a demolition and authorisation application, through to the corresponding recycling and disposal options. An overview is also given of the possible pollutants (e.g. asbestos, mineral fibres) and the corresponding protective measures.
- Dismantling of nuclear facilities. The aim of this course is to enable students to develop dismantling concepts and to select and use the necessary techniques and procedures, to implement the principles of authorisation and to draw up corresponding applications, to take into account and implement the requirements of the relevant laws.
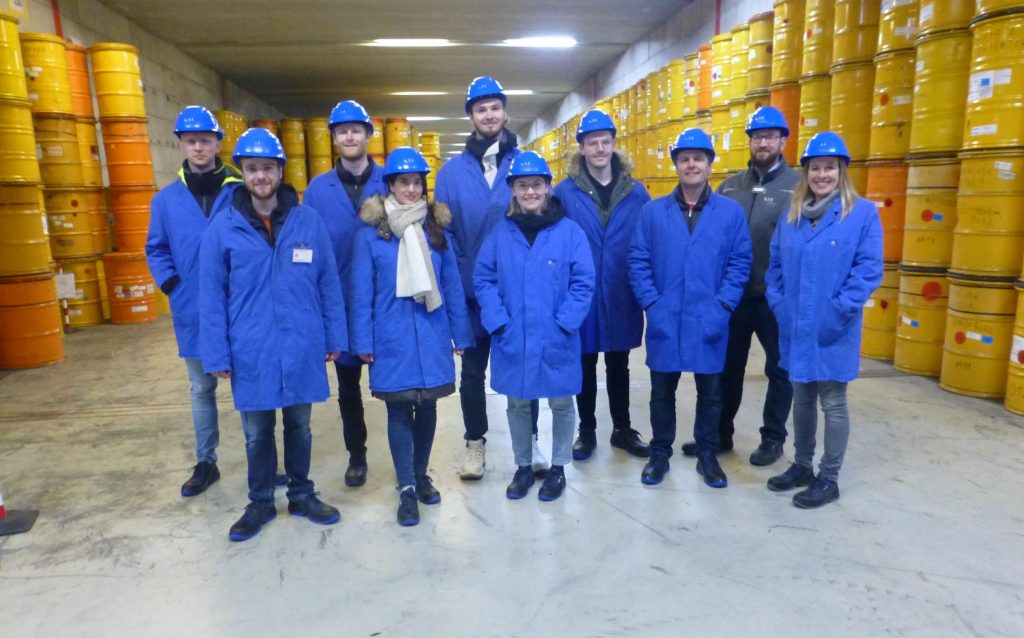
Visits to KTE - Kerntechnische Entsorgung Karlsruhe GmbH as part of the lecture "Dismantling nuclear facilities"
Finally, it should be noted that there are numerous opportunities for students at KIT to write a Bachelor's or Master's thesis as part of research work at the various institutes involved in the NUSAFE programme. In addition, doctoral theses are also offered at the institutes.
CONTACT
Karlsruhe Institute of Technology
KIT - Karlsruhe Institute of Technology
KIT Karlsruhe (@KITKarlsruhe) / X (twitter.com)
(27) KIT Karlsruhe Institute of Technology - YouTube
Institute for Neutron Physics and Reactor Technology (INR)
Phone: +49 721 608-22551
Institute for Applied Materials (IAM)
Phone: +49 721 608-24815
Institute for Nuclear Waste Management (INE)
Phone: +49 721 608 22231
Institute for Technology and Management in Construction (TMB)
Phone: +49 721 608-46546
The Institute of Physical Chemistry and Radiochemistry at Mannheim University of Applied Sciences can look back on over 50 years of tradition. Originally founded as the Institute of Nuclear Technology and Radiochemistry, it has undergone a number of changes over the decades. Almost eight years ago, Prof. Dr Ulrich W. Scherer took over radiochemistry and established a working group of currently around 10 people who are involved in teaching and research.
Core task teaching
Our core task is to train students of chemical and process engineering and mechanical engineering using modern teaching methods in the fields of radiochemistry and radiation protection.
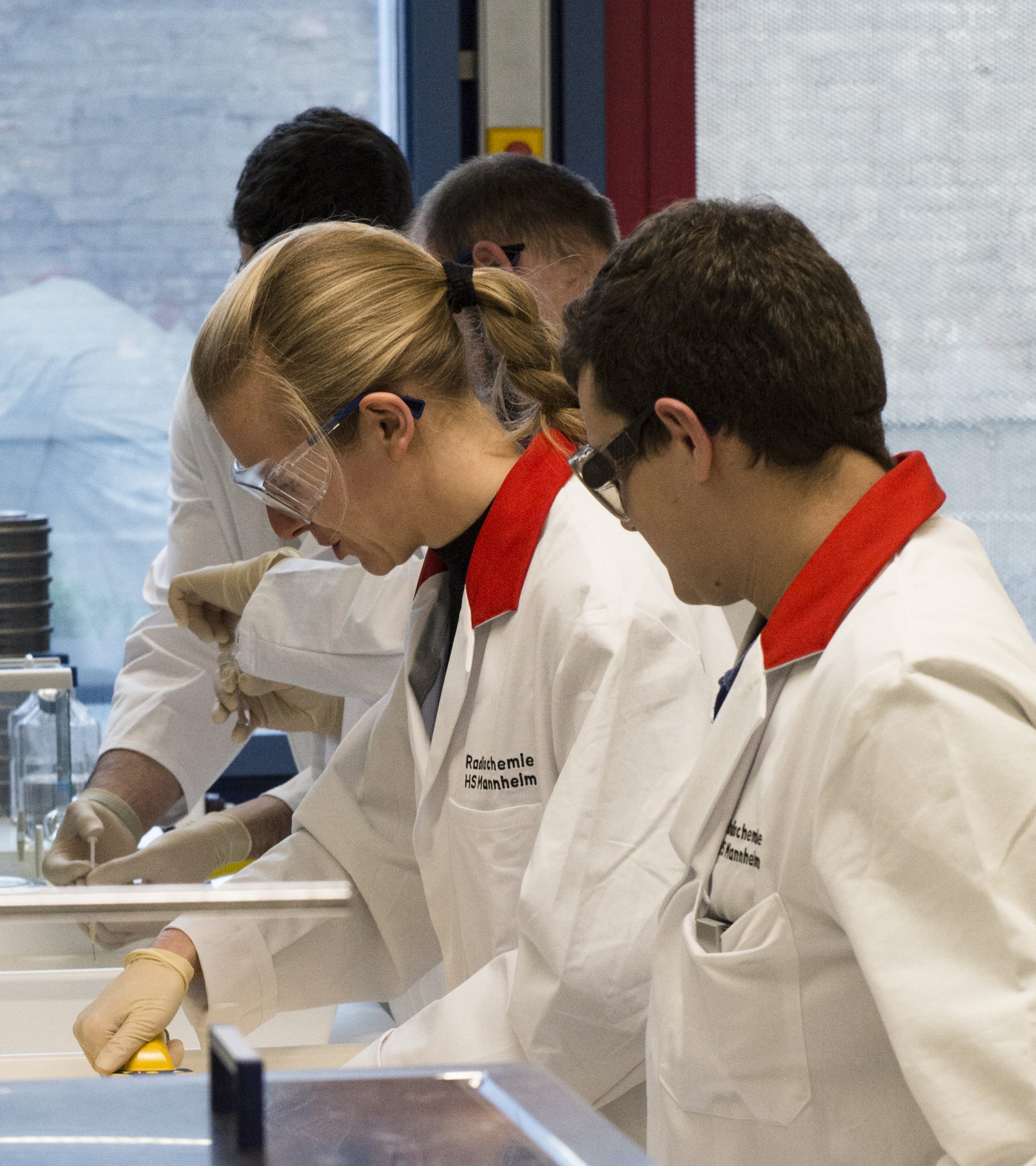
Students of the Mannheim University of Applied Sciences
In our understanding, radiochemistry encompasses all areas of handling open radioactive substances. In the lecture Radiochemistry The basics of radiochemical work are explained, right through to the applications of tracer technology with its many applications. An important chapter deals with the production of Nuclear fuels up to the Disposal of radioactive wastewhich is dealt with in a separate lecture. In addition, the practical handling of open radionuclides in a wide range of applications forms an important pillar of our training. Furthermore, we offer our students courses to acquire the Expertise in radiation protection to.
Our laboratories have additional handling licences and are also equipped with the optimum measurement technology for all types of ionising radiation. We also operate a hot cell and a 14 MeV neutron generator.
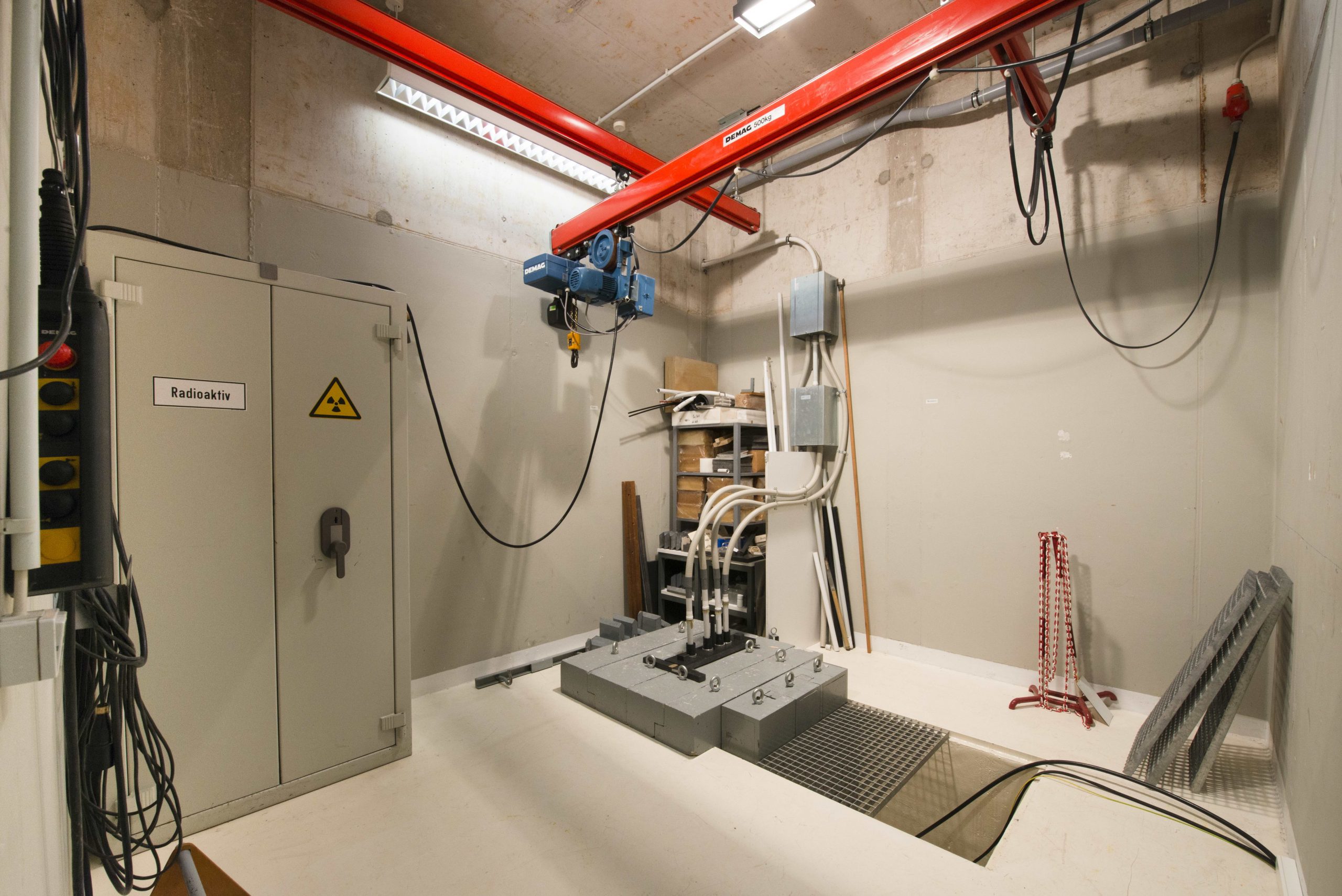
Irradiation room at the Manheim University of Applied Sciences
We are a member of the European university network CHERNE, which aims to improve teaching through co-operation and exchange and to give students from partner universities access to laboratories and large-scale equipment. Funded mainly by ERASMUS projects, we have been organising courses for almost twenty years, typically for around 20 students, dealing with nuclear technology, nuclear waste management, environmental radioactivity, but also with areas such as radionuclide production on cyclotrons.
Research and development
Our research activities are diverse. The applicability of our research results in practice is of great importance to us: the aim is to develop processes, procedures or devices with which an existing task can be (better) solved. For example, we have developed the prototype of an alpha detector on whose surface radioelements such as plutonium or americium can be selectively bound and spectrometrically measured with high yields. Its application can minimise the amount of personnel and time required to produce samples of alpha emitters and thus significantly increase sample throughput. We are currently developing this detector to market maturity for applications in decommissioning, NORM management and radiopharmaceuticals.
In a series of co-operation projects with companies based in the Rhine-Neckar metropolitan region, we have developed various projects for the Dismantling investigated. For example, an electrochemical process for sampling was developed and the use of pulsed high-power lasers for the decontamination of building materials was analysed both technically and economically. It is a particular sense of achievement to see the methods we have investigated being used in the plants.
In May of this year, we were able to record another success: As part of FORKA, we set up a junior research group under the leadership of Dr Lotte Lens, which is working on the Characterisation and decontamination of irradiated reactor graphite employed. Since then, we have taken on three PhD students who are preparing their work. We are currently waiting for the delivery of the newly procured larger devices for this ambitious research project.
Recently, we have been approached by international cooperation partners wishing to utilise our experience in the field of nuclear power plant chemistry. Such collaborations would be very important in terms of maintaining expertise, also in order to be able to evaluate the operation of nuclear power plants in our neighbouring countries, but also in other parts of the world. Due to our capacity utilisation, such projects are only possible through further expansion of the Group.
Further training
Another mainstay of our institute is our training programmes. Due to the well-known problems in recruiting specialists, we are now in our fourth year of offering certificate courses for beginners in the field of Dismantling and disposal to. In addition to companies based in the metropolitan region, participants are increasingly coming from other regions of Germany. The spectrum covers the scientific and engineering fundamentals of nuclear technology, but also special topics such as building clearance or radioactive waste disposal. Specialised courses also deal with nuclear measurement technology.
In recent years, we have also been able to organise a basic course for the approximately 70 new employees of the State Ministry of the Environment.
One possible further development would be the establishment of a master's degree programme in dismantling and waste disposal. Due to our framework conditions, this is only possible as a part-time degree programme. However, this requires relevant support from the state and industry. The offers promised so far are not yet sufficient to start this ambitious project.
Outlook
We are operating in a difficult environment: the universities of applied sciences are significantly less well funded by their sponsors than the universities, although the areas of responsibility have converged considerably. For example, research has been an established task for around 30 years, but the necessary resources (staff, space, equipment, funding) have not yet been provided. With teaching loads that are more than twice as high as those of university colleagues, this means a considerable amount of additional work. In addition, even though we have now been granted the right to award doctorates, our academic training is not primarily aimed at training doctoral students. As a result, the federal government's funding measures often fall short for us or do not apply at all, as they only relate to research funding for projects carried out by doctoral students due to the division of responsibilities between the federal and state governments. We would like to see a change in procedures that takes our structure into account.
Otherwise, I can only agree with the opinion of many colleagues: Maintaining expertise in nuclear engineering is only possible if the remaining professorships and chairs are retained. It is still possible to convince students of these interesting and attractive fields of work. The establishment of a cross-university academy is certainly a measure worth considering to pool expertise.
Prof Dr Ulrich W. Scherer
Head of the Institute of Physical Chemistry and Radiochemistry and Radiation Protection Officer at Mannheim University of Applied Sciences
Mannheim University of Applied Sciences - Mannheim University of Applied Sciences (hs-mannheim.de)
Mannheim University of Applied Sciences: Overview | LinkedIn
University